Taking Steps Forward—Advancing Conventional Methods of Medical CT Imaging
Since the 1970s, the field of medicine has relied on the imaging technique of X-ray computed tomography (CT) to visualize soft tissues of the body, bone structures, and to assist in the diagnosis of a myriad of diseases.1,2 A CT scanner creates a beam of X-rays from an X-ray source aimed at a patient and recognized by rows of detectors, producing multiple images (“slices”) that are processed by the machine’s computer, generating cross-sectional images.3 Beyond diagnostic purposes, this technology is used to select suitable treatment options for patients. The images produced by CT scanners spans various medical disciplines. CT scanners are vital tools for imaging of the head, lung, heart, abdomen, pelvis, and extremities. Despite the wide use of these instruments, there have been limitations to this technology. To combat the technical challenges of poor plane resolution and protracted scanning, scientists have made improvements to conventional methods of CT imaging.4-6 Since the late 1990s, CT has evolved to encompass “multi-detector” (MDCT) equipment capable of acquiring slices at a faster rate and with wider coverage.
Recent innovations to MDCT are the detector efficiency, the addition of a second X-ray beam (dual energy/dual source), a higher kilovoltage peak on the X-ray source, and a greater output of X-ray photons along with improved reconstruction algorithms.7 These innovations allow for higher spatial resolution, faster imaging, higher contrast resolution, and a larger diversity of application(s) of this technology. Greater contrast and improved spatial resolution facilitates the delineation of previously undetected anatomical details or the detection of function (i.e., tissue perfusion or regional ventilation in the lungs) with lower amounts of injected contrast material. Additional advantages of the dual source configuration include the elimination of shading and streaking, which are imaging artifacts commonly associated with patient motion (e.g., breathing).4 Reducing artifacts is significant because these can interfere with an instrument’s performance and affect the accuracy of image interpretation, thus delaying patient examination. Faster imaging enables the extension of CT in service of patients, such as infants that cannot endure long imaging sessions.
The primary challenge with CT is achieving high-resolution images while continuing to reduce levels of ionizing radiation. Early in the history of CT imaging, sharper CT images were attained through longer exposures and multiple scans. The combined multi-detector system and dual source technology addresses this challenge. Dual source MDCT coupled with the improvements to the X-ray delivery system and more efficient detectors affords better safety to patients than earlier CT designs. Increased safety is achieved because of its ability to accomplish higher quality images at lower radiation doses, 7,8 thereby minimizing the risk of carcinogenesis due to radiation exposure levels observed with conventional CT.
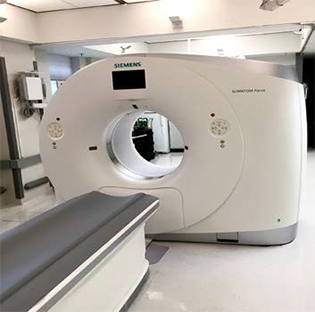
Dual source/dual energy MDCT scanners have redefined the imaging standards in biomedical research, revolutionized CT technology, and contributed greatly to preventative medicine by informing the biomedical community of the potential risk factors for lung diseases, such as Chronic Obstructive Pulmonary Disease (COPD), cancer, as well as cardiac- and orthopedic-related ailments.4 Despite the observable advantages of dual source/dual energy MDCT scanners, access to this technology, especially for research development, is limited for certain institutions.
Access to dual source/dual energy MDCT scanners enable scientists to apply imaging methods to novel medical fields and to image certain groups of patients not previously contemplated. The National Institutes of Health’s (NIH) Office of Research Infrastructure Programs (ORIP) is committed to offering much-needed access to instruments that otherwise may not be available to many scientists. ORIP’s S10 Instrumentation Grant Program in 2015 awarded a dual energy MDCT scanner, the “SOMATOM Force” to Dr. Eric Hoffman, Director of the Advanced Pulmonary Physiomic Imaging Laboratory at the University of Iowa.
This scanner (Siemens Healthcare, Germany) is being used to study areas related to pulmonology, orthopedics, dentistry, atherosclerosis, and cardiology. Dr. Hoffman adds, “the most important aspect (of the physiomic laboratory) is to allow the development of imaging methodologies to allow us to look inside the ‘never open chest’ and gain an understanding of the link between the structure of the lung and the function of the lung, and what goes wrong under pathologic states.”
The core application of this dual source/dual energy MDCT are two multi-institutional observational projects to study COPD, which is a disease of pathological heterogeneity that affects more than 3 million individuals in the United States annually. These projects are assisting scientists to discover biological markers that may predict patient-specific treatment approaches, which aims toward crafting personalized care for ailments, such as emphysema.9-11 The scanner permits for the development of higher resolution/lower X-ray dose imaging protocols that may be used by scientists studying various aspects of lung physiology.
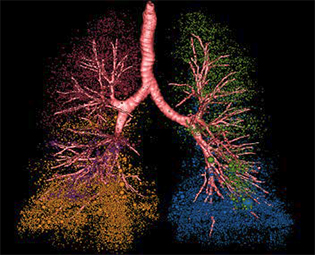
Through the Iowa Comprehensive Lung Imaging Center Consortia, several scientists are collaborating to conduct lung imaging clinical trials. An observational study12 comprising more than 2,000 current or former smokers utilized imaging methodologies developed through the S10 grant funded facility. In this study, investigators discovered that approximately half of smokers have respiratory symptoms mirroring COPD despite the absence of the hallmark feature of this disease, pulmonary obstruction. Remarkably, these results suggest that the clinical definition of COPD should be adjusted.
Through the S10 grant mechanism, the dual source/dual energy MDCT scanner has supported a diverse amount of NIH-funded research areas at a number of institutions. The low radiation dose/high resolution capability of the dual energy MDCT has allowed Dr. Punam Saha’s laboratory to successfully image distal regions of the human tibia to better characterize structures that correlate to bone strength.13 The current interest is to identify which COPD sufferers might be more susceptible to spinal fractures and the risk may be reflected in changes to distal tibia structure. In another study,14 the fetal lung development gene, Cadherin EGF LAG Seven-Pass G-Type Receptor 1, was identified as a potential sex-specific genetic risk factor for COPD development. This result presents a tremendous advancement toward identifying preventative care for COPD in certain patients. Further highlighting the diversity of research made possible by the use of this instrument, the laboratory of Dr. Steven Rowe (University of Birmingham, Alabama), taking advantage of patients studied in a multi-center study imaged, in part, at the S10 grant funded facility, is researching the role of a novel glycopolymer in improving abnormal mucus levels and bacterial biofilms in the human body. The past and present use of this instrument has resulted last year in the publication of dozens of research articles and abstracts from a variety of institutions demonstrating the tremendous impact of the improved imaging technology made available to the biomedical community through the S10 grant.
It is predicted that the innovative technology and long-term investment of advanced MDCT scanners will enhance the understanding of disease processes and continue to provide a much-needed resource for guiding medical providers in offering prevention strategies and earlier diagnoses.
References
1 Littleton, JT. Conventional tomography. A history of the radiological sciences. American Roentgen Ray Society. Retrieved 11 January 2014.
2 Beckmann E. CT scanning the early days. The British Journal of Radiology. 2006;79(937):5–8.
3 Hounsfield G. Computerized transverse axial scanning (tomography): part 1: description of system. The British Journal of Radiology. 1972;46:1016–1022.
4 Karçaaltıncaba M, Aktaş A. Dual-energy CT revisited with multidetector CT: review of principles and clinical applications. Diagnostic and Interventional Radiology. 2011;17(3):181–194.
5 Graser A, Johnson TR, Chandarana H, Macari M. Dual energy CT: preliminary observations and potential clinical applications in the abdomen. European Radiology. 2009;19(1):13–23.
6 Coursey CA, Nelson RC, Boll DT, Paulson EK, Ho LM, Neville AM, et al. Dual-energy multidetector CT: how does it work, what can it tell us, and when can we use it in abdominopelvic imaging? Radiographics: A Review Publication of the Radiology Society of North America, Inc. 2010;30(4):1037–1055.
7 Newell JD Jr, Fuld MK, Allmendinger T, Sieren JP, Chan KS, Guo J, Hoffman EA. Very low-dose (0.15 mGy) chest CT protocols using the COPDGene 2 test object and a third-generation dual-source CT scanner with corresponding third-generation iterative reconstruction software. Investigative Radiology. 2015;50(1):40–45.
8 Hammond E, Sloan C, Newell JD Jr, Sieren JP, Saylor M, Vidal C, et al. Comparison of low- and ultralow-dose computed tomography protocols for quantitative lung and airway assessment. Medical Physics. 2017; Jun 28. doi: 10.1002/mp.12436.
9 Regan EA, Hokanson JE, Murphy JR, Make B, Lynch DA, Beaty TH, et al. Genetic epidemiology of COPD (COPDGene) study design. COPD. 2010;7(1):32–43.
10 Couper D, LaVange LM, Han M, Barr RG, Bleecker E, Hoffman EA, et al. Design of the Subpopulations and Intermediate Outcomes in COPD Study (SPIROMICS). Thorax. 2014;69(5):491–494.
11 Sieren JP, Newell JD Jr, Barr RG, Bleecker ER, Burnette N, Carretta EE, et al. SPIROMICS Protocol for Multicenter Quantitative Computed Tomography to Phenotype the Lungs. American Journal of Respiratory and Critical Care Medicine. 2016;194(7):794–806.
12 Woodruff PG, Barr RG, Bleecker E, Christenson SA, Couper D, Curtis JL, et al. Clinical significance of symptoms in smokers with preserved pulmonary function. New England Journal of Medicine. 2016;374 (10):1811–1821.
13 Saha PK, Liu Y, Chen C, Jin D, Letuchy EM, Xu Z, et al. Characterization of trabecular bone plate-rod microarchitecture using multirow detector CT and the tensor scale: algorithms, validation, and applications to pilot human studies. Medical Physics. 2015;42(9):5410–5425.
14 Hardin M, Cho MH, Sharma S, Glass K, Castaldi PJ, McDonald ML, et al. COPDGene and evaluation of COPD longitudinally to identify predictive surrogate end-points investigators. Sex-based genetic association study identifies CELSR1 as a possible chronic obstructive pulmonary disease risk locus among women. American Journal of Respiratory Cell and Molecular Biology. 2017;56(3):332–341.