Mouse Research Yields New Insights on Mendelian Disease Genetics
Gregor Mendel’s work in the 19th century laid the foundation for our current understanding of heritability—the ways in which phenotypes (i.e., an individual’s observable traits) are passed through generations. Since that time, researchers have determined that many traits and diseases reflect the interplay of multiple genes and therefore are much more complex than those in Mendel’s initial reports. A significant subset of human disorders, however, is indeed caused by a single genetic mutation. Well-known examples of Mendelian diseases include cystic fibrosis, sickle cell disease, and Duchenne muscular dystrophy. Although the genetic mechanism of these diseases might appear simple, many of the underlying mutations remain unknown.
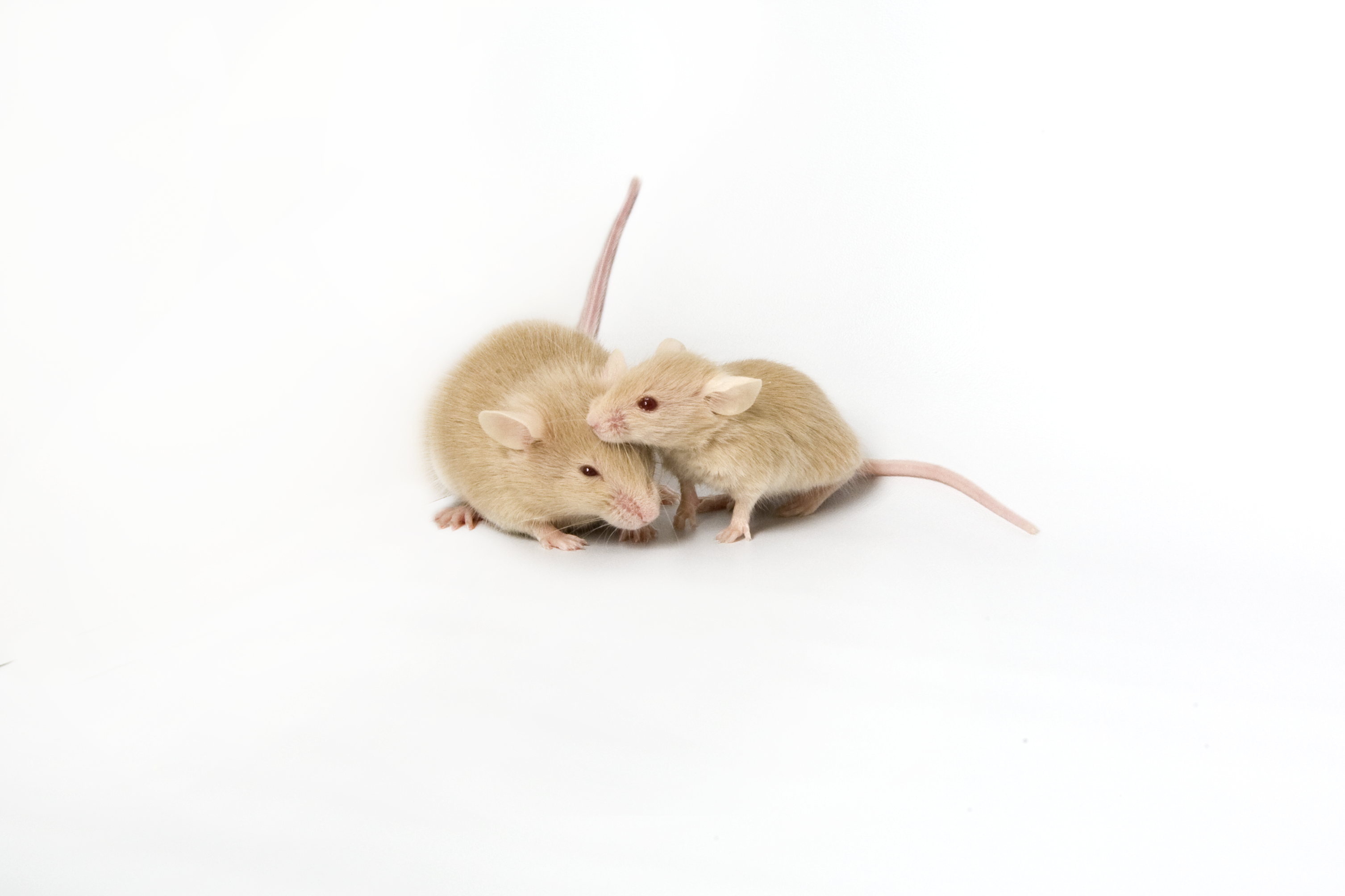
Dr. Laura Reinholdt, Associate Professor at The Jackson Laboratory (JAX), explained that many of the genetic mutations present in Mendelian disorders—such as structural variants—are challenging to detect accurately. Additionally, pedigree data often are lacking in clinical cases, making it difficult to identify new Mendelian disease alleles in human patients. Recent advances in genomic technologies, however, might provide the key to understanding the basis of Mendelian diseases. Dr. Reinholdt receives funding from ORIP (R24OD021325) to provide resources in this area to the research community. This work involves conducting studies of naturally occurring Mendelian mutations in mice; the project also links discovered genetic and phenotyping information with human databases to help identify the causes of human diseases (Figures 1 and 2). The mutant animals also can serve as models to identify and test treatment options.
“We know that different types of mutations cause genetic disease, and we’re getting better and better at finding the more elusive structural mutations that involve larger regions of DNA. But it’s still not reduced to practice,” Dr. Reinholdt stated. “Another issue is not having enough patient data to build a case for a specific gene—especially where there are novel genes or alleles involved in the disease.”
For years, researchers have faced technological limitations in detecting all types of naturally occurring genetic variants, Dr. Reinholdt explained. Most high-throughput technologies are based on short-read sequencing, which is useful for identifying single nucleotide variants or small gene insertions and deletions but often falls short in detecting larger structural variants (e.g., large insertions, translocations, duplications). Recent advances have allowed researchers to apply long-read sequencing techniques that can provide researchers with a greater amount of useful data for analysis.
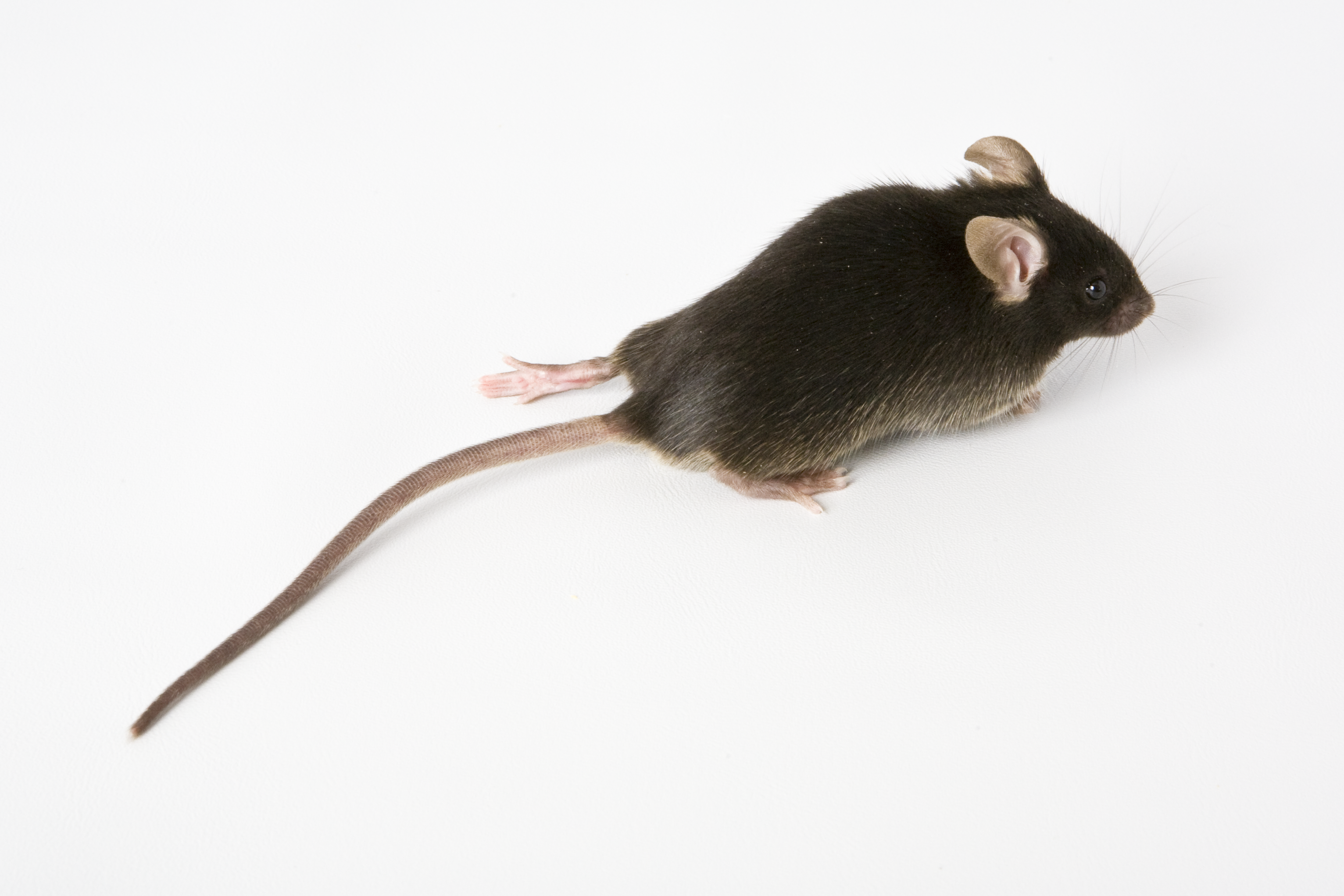
At JAX, Dr. Reinholdt’s group is applying these technologies to characterize the genetic nature of Mendelian mutations in mice. For more than 100 years, researchers have been using mice to gain a better understanding of disease. Researchers study genetic traits in mice using two approaches: forward genetics (i.e., determining the underlying genetic basis of a visible phenotype) and reverse genetics (i.e., targeting a gene by engineering and assessing the resulting phenotype). Dr. Reinholdt explained that her program at JAX takes advantage of a classic forward genetics technique—the researchers characterize naturally occurring mutations that are present within JAX’s extensive mouse population
“In biomedical research, we always get excited about doing new things. When we started engineering the mouse genome, we thought we’d never need to use spontaneous mouse mutants again,” Dr. Reinholdt reflected. “Yet, in engineering, it is still hard to predict which inbred strain background will yield a clinically relevant phenotype, and increasingly we recognize that inbred strain backgrounds themselves are not always appropriate. Moreover, some types of mutations, like structural mutations, are still hard to engineer. This highlights the advantage of forward genetics. If we happen to find a mouse strain that already has a clinically relevant phenotype, we can use forward genetics to find out what the underlying genetics tells us. This is still an important process.”
JAX, as one of the world’s largest laboratory mouse suppliers, conducts a comprehensive quality control process to monitor emergent phenotypes in its colonies. Among the millions of mice tested, occasional variations in phenotype can occur—due to random genetic mutations that arise naturally within the colonies, in much the same way that new disease-causing mutations arise in human populations. Researchers have exploited these “spontaneous mutants” to understand and model human diseases for more than 100 years, and JAX has supported these efforts since its founding in 1929. With new genomic technologies, this process is faster and more efficient than ever.
As an example, Dr. Reinholdt’s team discovered a mutation within the collection that seemed to affect the animal’s gait and body size. Unbeknownst to the JAX team, the mutation was in a gene that was being investigated by a team of clinical researchers at Cincinnati Children’s Hospital Medical Center. Dr. Taosheng Huang’s group was studying a group of clinical patients who appeared to present with mitochondrial disorders and optic atrophy. At the time, the genetic basis of the patients’ symptoms was unknown. Because JAX continuously shares its findings with clinical centers and other research projects, the two teams connected, forming a collaboration to better understand the disorder in both clinical and laboratory contexts. Working together, they determined that the disorder was caused by a mutation in the mitochondrial ferredoxin reductase gene (Fdxr). This insight has allowed faster characterization of the disease and development of potential therapeutics for patients.1–3
Dr. Reinholdt explained that her team’s collaborative approach is crucial for developing robust models with face validity (i.e., the degree to which an animal model replicates a phenotype in humans). Her team now is developing a database of mouse variants that will allow data sharing across research projects. Researchers can upload their data through this tool to help enable discovery of unique and new variants that have not been identified previously. Dr. Reinholdt’s team regularly contributes to the European Nucleotide Archive—which allows genetic comparisons across model organisms—as well as JAX’s Mouse Genome Informatics Database, the Rare Disease Translational Center, and the Centers for Mendelian Genomics. Dr. Reinholdt emphasized that by gaining a better understanding of Mendelian traits, the research community can gain a better understanding of many different genetic disorders. JAX’s efforts in discovering and characterizing these mouse strains contributes to the distribution of validated animal models for human diseases.
“With our resource, it’s not just the mouse models that come out of it—it’s also pipelines and data that we hope will really empower that mouse genetics community as they are working toward leveraging different types of sequencing technologies to find which mutations underlie clinically relevant phenotypes,” Dr. Reinholdt emphasized. “And that could extend beyond just Mendelian disease—it could apply to complex disease phenotypes in mice as well.”
References
1 Yang L, Slone J, Zou W, et al. Systemic delivery of AAV-Fdxr mitigates the phenotypes of mitochondrial disorders in Fdxr mutant mice. Mol Ther Methods Clin Dev. 2020;18:84–97. doi:10.1016/j.omtm.2020.05.021.
2 Slone JD, Yang L, Peng Y, et al. Integrated analysis of the molecular pathogenesis of FDXR-associated disease. Cell Death Dis. 2020;11(6):423. doi:10.1038/s41419-020-2637-3.
3 Slone J, Peng Y, Chamberlin A, et al. Biallelic mutations in FDXR cause neurodegeneration associated with inflammation. J Hum Genet. 2018;63(12):1211–1222. doi:10.1038/s10038-018-0515-y.